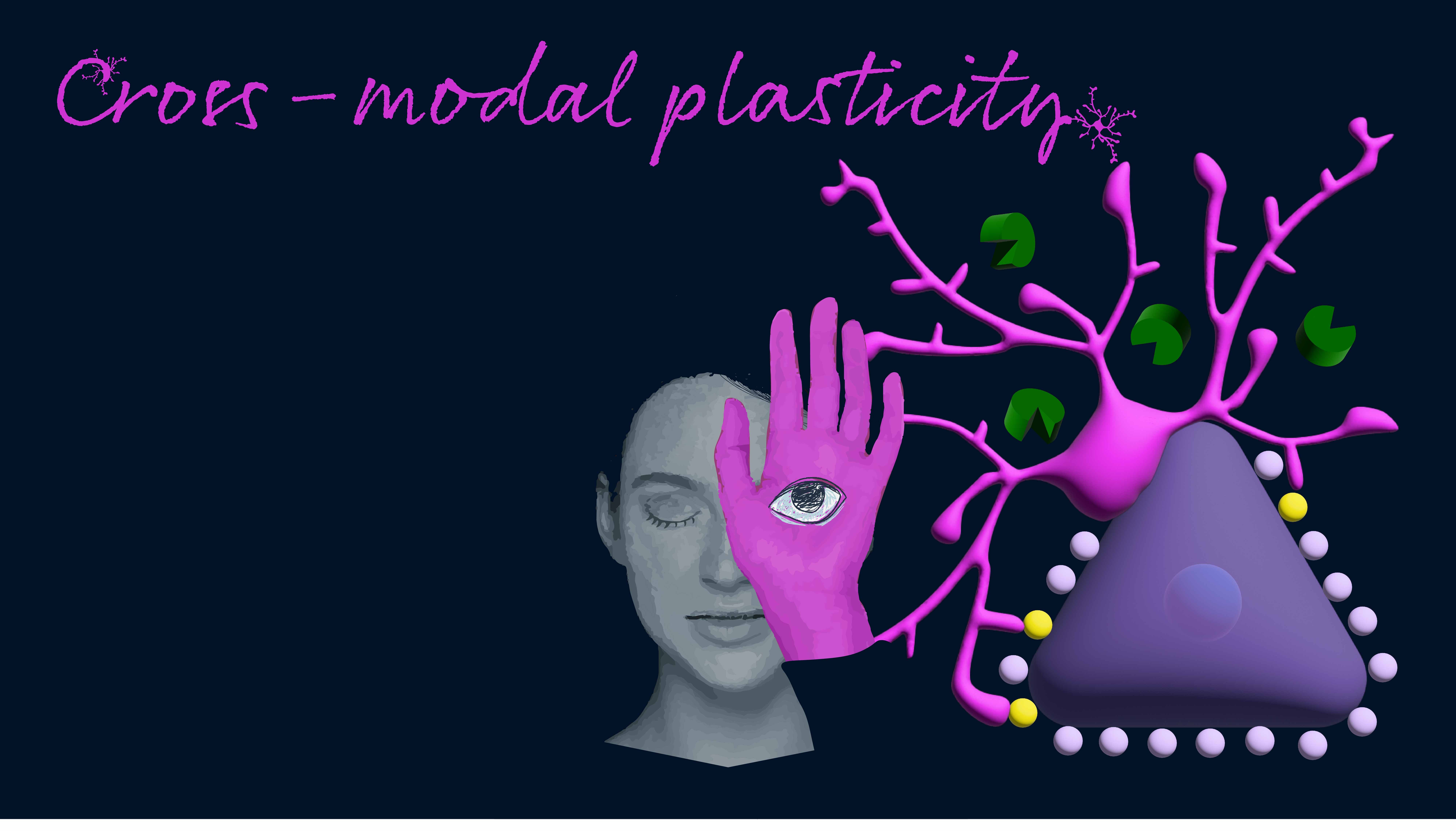
A research team led by Akari Hashimoto and Professor Hiroaki Wake of the Department of Anatomy and Molecular Cell Biology, Nagoya University Graduate School of Medicine, has uncovered the mechanism behind the improved tactile perception after early vision loss.
We utilize various sensory information such as sight, touch, and hearing to perceive our surroundings. Visual information is mainly processed in the visual cortex located in the occipital lobe, somatosensory information in the somatosensory cortex located in the parietal lobe, and auditory information in the auditory cortex located in the temporal lobe. However, when we lose our sight, the visual cortex does not become dysfunctional. Instead, the visual cortex begins to process the other types of sensory information, such as auditory and somatosensory information. This phenomenon is called "cross-modal plasticity", resulting in the increase ability of the “sensitivity” to touch and hearing. This phenomenon has been widely known since the 1990s. When congenitally blind people read braille (in other words, getting somatosensory stimulation), their visual cortex is activated in addition to the somatosensory cortex. However, the mechanism has not fully been understood. The research group shed light on it, revealing the microglial contribution on cross-modal plasticity.
Our brain consists of more than 100 billion neurons and as many as one trillion of glial cells. Neurons transmit and process information using electrical signals, while glial cells play roles in forming and regulating neural circuits, and in nourishing neurons. It has been recently known that microglia, sole immune cells in the brain, constantly extending and retracting its processes, and monitor, form, and remove synapses. Microglia thus contribute to the formation and refinement of neural circuits during development and learning, as well as the reorganization of neural circuits. In particular, the effect of microglia on excitatory synapses has been well discussed in this context. However, the involvement of microglia in inhibitory synapses has not yet been fully understood.
In this study, the team used various approaches such as two-photon in vivo calcium imaging, electron microscopy, electrophysiology, and molecular biology, to reveal the mechanism that the higher visual cortex responds to somatosensory information from whisker after visual deprivation, resulting in improved sensory discrimination ability. When mice are visually deprived early in life, microglia in the higher visual cortex remove inhibitory synapses, thereby releasing the inhibitory neural circuits that suppress the inherited pathway from the somatosensory cortex to the higher visual cortex. As a result, neurons in the higher visual cortex become responsive to whisker stimulation, and this leads to improved tactile perception. Furthermore, the group demonstrated that microglia remove inhibitory synapses by dissolving the extracellular matrix using matrix metalloproteinase 9.
Recently, it has been known that in healthy individuals, not only visual but also auditory and somatosensory stimuli can influence the neural activity of the higher visual cortex. In this sense, the higher visual cortex is being considered to play an important role in sensory integration. The group advocated the somatosensory information processing mechanism in the higher visual cortex and its control by microglia in this study, and it will prompt to find new mechanisms of the integration and discrimination of multiple sensory modalities in the brain in the near future. Furthermore, considering previous papers report a reduction in inhibitory synapses and impaired sensory integration in autism spectrum disorder (ASD) and schizophrenia, the mechanism they found might become a new target for treatment of it.
This study was performed with the cooperation of Professor Takeshi Yagi and Assistant Professor Etsuko Tarusawa and Nanami Kawamura in KOKORO-Biology Group, Graduate School of Frontier Biosciences, Osaka University, Professor Nobuhiko Ohno in Department of Anatomy, Jichi Medical University, and international collaboration with Associate Professor Andrew J Moorhouse of School of Biomedical Sciences, The University of New South Wales, and Anne Schaefer in Icahn School of Medicine at Mount Sinai.
This work was published in Cell Reports on April 21, 2023. |
Key Points
-
The higher visual cortex of congenitally blind mice exhibits increased neural activity in response to somatosensory stimulation, resulting in improved tactile perception.
-
After visual deprivation, microglia remove the inherited circuit that suppresses the pathway from somatosensory cortex to the higher visual cortex, and increase neural response to tactile stimuli.
-
The removal of this inhibitory neural circuits is induced by microglia dissolving the extracellular matrix with matrix metalloproteinase 9 and stripping inhibitory synapses.
1. Research Background
We utilize various sensory information such as sight, touch, and hearing to perceive our surroundings. Visual information is mainly processed in the visual cortex located in the occipital lobe, somatosensory information in the somatosensory cortex located in the parietal lobe, and auditory information in the auditory cortex located in the temporal lobe. However, when we lose our sight, the visual cortex does not become dysfunctional. Instead, the visual cortex begins to process the other types of sensory information, such as auditory and somatosensory information. This phenomenon is called "cross-modal plasticity", resulting in the increasement of the sensitivity to touch and hearing. This phenomenon has been widely known since the 1990s. When congenitally blind people read braille (in other words, getting somatosensory stimulation), their visual cortex is activated in addition to the somatosensory cortex. A similar phenomenon has been observed in mice, where the visual cortex of monocular deprived mice is activated in response to whisker stimulation (note that whiskers are an important source of somatosensory information for mice, akin to the role of fingers in humans). However, the mechanism has not fully been understood.
2. Research Results
The research group found the mechanism of cross-modal plasticity, revealing the microglial involvement in its process. It has been recently known that microglia, one type of the glial cells, constantly extending and retracting its processes, and monitor, form, and remove synapses and contribute to the formation and refinement of neural circuits during development and learning, as well as the reorganization after dysfunction. In this study, the team used various approaches such as two-photon in vivo calcium imaging, electron microscopy, electrophysiology, and molecular biology, to reveal the mechanism that the higher visual cortex comes to respond to somatosensory information from whisker with the help of microglia after visual deprivation, resulting in improved sensory discrimination ability. When mice are visually deprived early in life, microglia in the higher visual cortex remove inhibitory synapses, thereby releasing the inhibitory neural circuits that suppress the inherited pathway from the somatosensory cortex to the higher visual cortex. As a result, neurons in the higher visual cortex become responsive to whisker stimulation, and this leads to improved tactile perception. Furthermore, the group demonstrated that microglia remove inhibitory synapses by dissolving the extracellular matrix using matrix metalloproteinase 9.
(1) The neuronal pathway from the primary somatosensory cortex to the higher visual cortex responses to whisker stimulation.
Firstly, the research group discovered the existence of axonal projections from the primary somatosensory barrel cortex, which processes whisker information in mice, to a subset of regions within the higher visual cortex, called the AL area. To analyze whether this cortical pathway transmit whisker stimulation information to the higher visual cortex, the researchers observed the activity of these axons using the technique of in vivo two-photon calcium imaging. For visual deprived model, the group used mice that underwent monocular eyelid suture at two weeks of age, shortly after eye-opening. The group performed axonal calcium imaging of normal-sighted and visual deprived mice, and both groups exhibited strong axonal activity in response to whisker stimulation in the same extent. (Figure 1).
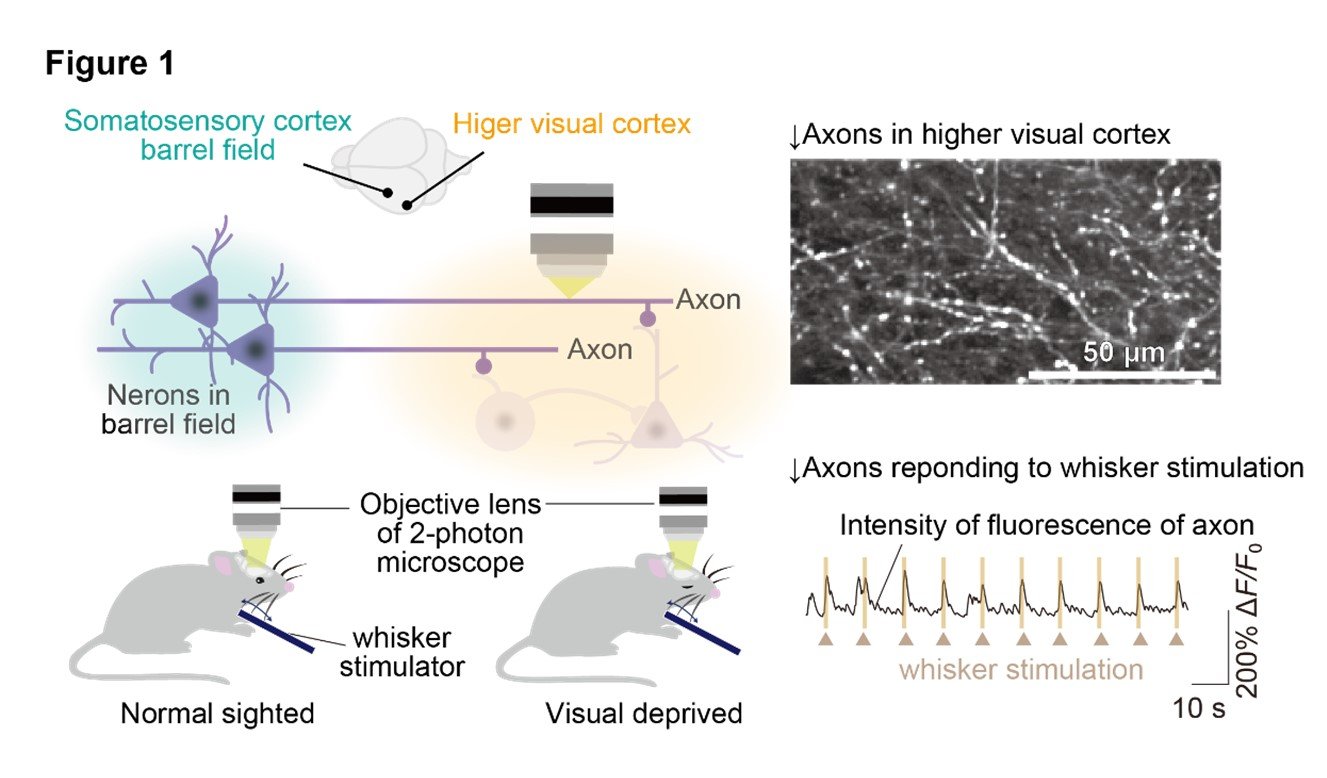
(2) Visual deprivation leads to an increase in neural activity of the higher visual cortex under whisker stimulation.
We conducted in vivo calcium imaging to observe the neural activity in the higher visual cortex in response to whisker stimulation. Visual deprived mice showed increased neural activity in response to whisker stimulation compared to normal mice. Furthermore, we found that the increased neural activity after visual deprivation was abolished by removing microglia using a drug called PLX3397.
These results suggest that microglia-dependent reorganization of neural circuits in the higher visual cortex is induced after visual deprivation. Interestingly, in normal mice, the neural activity during whisker stimulation was suppressed compared to spontaneous activity. (Figure 2).
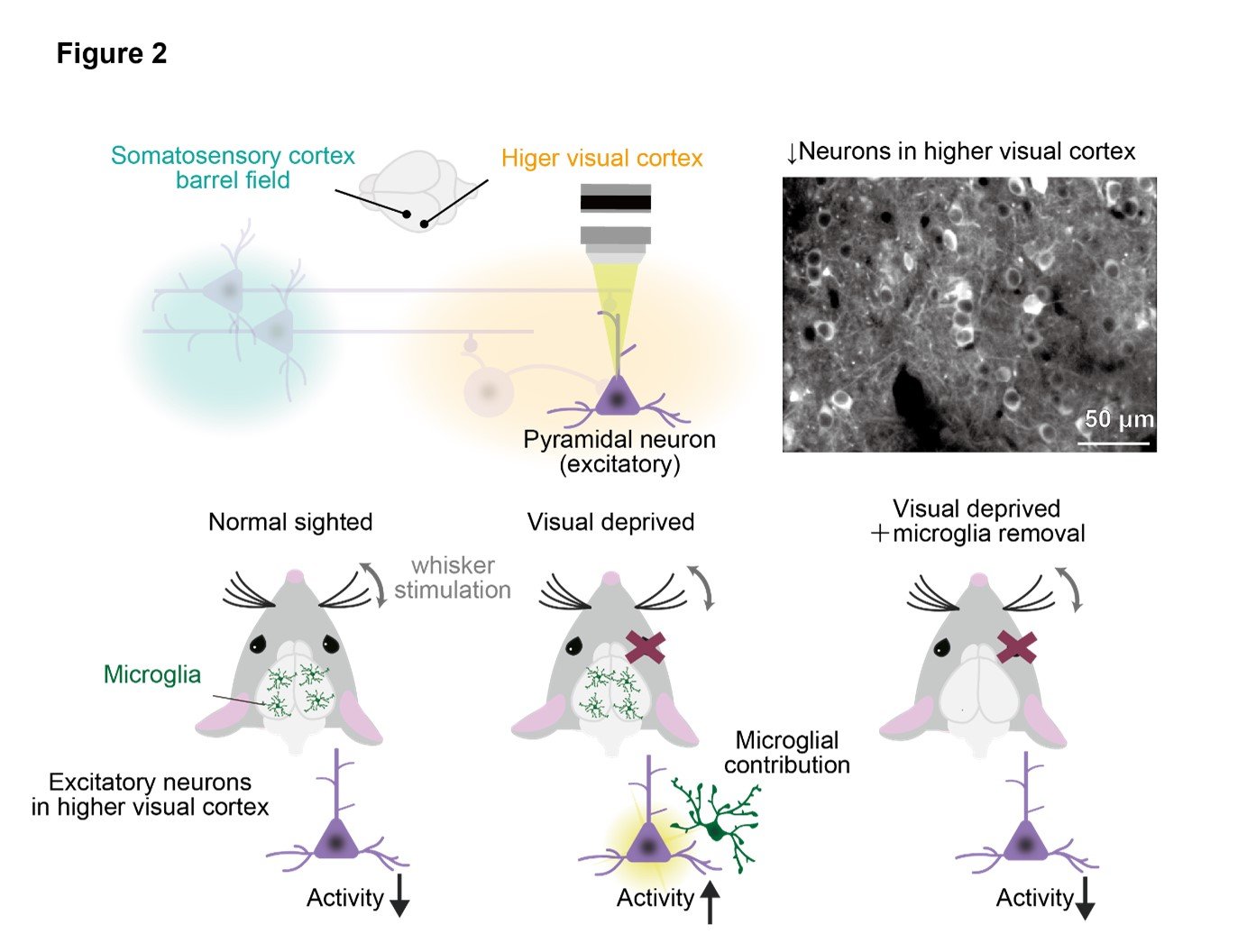
(3) Microglia dissolve the extracellular matrix and strip inhibitory synapses, leading to a reduction in inhibitory inputs to excitatory neurons after visual deprivation.
Based on the previous results, it was hypothesized that microglia play a role in the neural circuit reorganization in the higher visual cortex after visual deprivation. The research group therefore focused on the involvement of microglia in synaptic plasticity after visual deprivation. Five days after visual deprivation, microglia engulf inhibitory synapses, resulting in reduction of inhibitory synapses. Furthermore, microglia closely envelop the cell bodies of excitatory pyramidal cells and strip away inhibitory synaptic inputs derived from parvalbumin-positive cells. Electrophysiology also confirmed a decrease in inhibitory input after visual deprivation. By forcibly expressing channelrhodopsin in the barrel cortex and recording responses to photo-stimuli in the higher visual cortex, excitatory pyramidal cells and inhibitory parvalbumin-positive neurons receiving projections from the barrel cortex were identified. The strength of their synaptic connections was confirmed by paired recording, which revealed a decrease in synaptic input from parvalbumin-positive neurons to pyramidal cells after visual deprivation, and this phenomenon was abolished upon removal of microglia. Additionally, an increase in expression of matrix metalloproteinase 9 was observed in microglia after visual deprivation, suggesting that microglia infiltrate the synaptic cleft by digesting the extracellular matrix surrounding neuronal cell bodies. Indeed, administration of a matrix metalloproteinase 9 inhibitor resulted in suppression of neural activity in response to whisker stimulation in the higher visual cortex. (Figure 3).
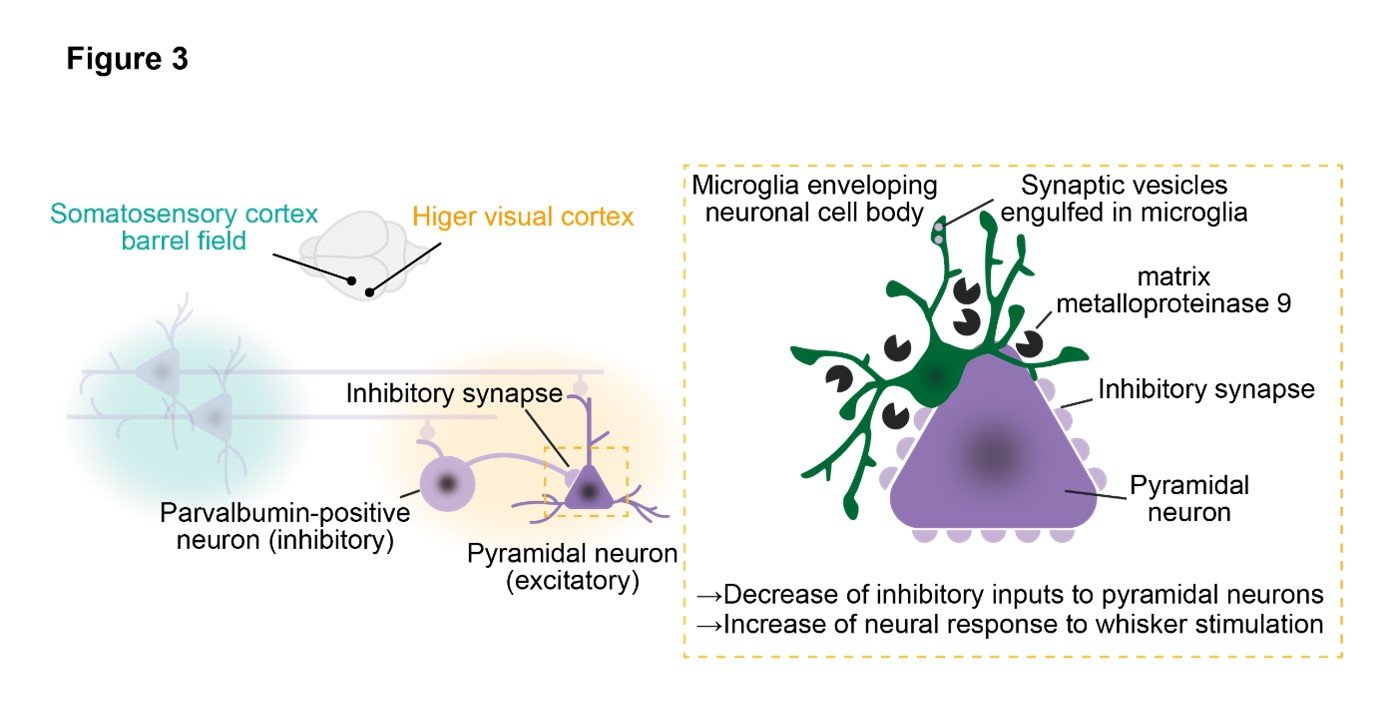
(4) Tactile perception improves in a neural activity-dependent manner of the higher visual cortex after visual deprivation,
To investigate whether the neural responses to whisker stimulation in the higher visual cortex after visual deprivation contribute to the improvement of tactile perception, the research group performed a sensory discrimination task where mice were rewarded when they correctly discriminate between a coarse and fine sandpaper presented randomly. The visual deprived mice showed faster learning compared to normal mice. However, when the neural activity in the higher visual cortex was locally suppressed with a drug called muscimol, the learning efficiency of visually deprived mice decreased. Additionally, visually deprived mice with microglia removal also showed a decrease in learning efficiency. These results suggest that microglia-mediated neural circuit remodeling in the higher visual cortex after visual deprivation contributes to the improvement of somatosensory ability.
3. Future Perspective
This study proved that visual deprivation induces microglial-mediated elimination of inhibitory synapses in the higher visual cortex, leading to enhanced neural activity and compensatory improvement of tactile perception. Recently, it has been known that in healthy individuals, not only visual but also auditory and somatosensory stimuli can influence the neural activity of the higher visual cortex. In this sense, the higher visual cortex is being considered to play an important role in sensory integration. The group advocated the somatosensory information processing mechanism in the higher visual cortex and its control by microglia in this study, and it will prompt to find new mechanisms of the integration and discrimination of multiple sensory modalities in the brain in the near future. Furthermore, considering previous papers report a reduction in inhibitory synapses and impaired sensory integration in autism spectrum disorder ASD and schizophrenia, the mechanism they found might become a new target for treatment of it.
4. Acknowledgements
This study was financially supported by the Astellas Foundation for Research on Metabolic Disorders; Grants-in-Aid for Scientific Research on Innovative Areas (19H04753, 19H05219, and 25110732 to H.W.); Grants-in-Aid for Transformative Research Areas (A) (20H05899 to H.W.); Fostering Joint International Research (B) (20KK0170 to H.W.); Grant-in-Aid for Scientific Research (B) (18H02598 and 21H02662 to H.W.); The Uehara Memorial Foundation to H.W. Additionally, it was supported by technical support platforms for promoting research of Advanced Bioimaging Support (JP16H06280), Takeda Science Foundation to J.N.; Grand-in-Aid for Transformative Research Areas (A) (21H05688 to I.T.); and JST CREST Grant Numbers JPMJCR1755 and JPMJCR22P6, Japan, to H.W.
5. Publication
ournal: Cell Reports
Title: Microglia Enable Cross-Modal Plasticity by Removing Inhibitory Synapses
Authors: Akari Hashimoto1, Nanami Kawamura2, Etsuko Tarusawa2, Ikuko Takeda1, Yuki Aoyama1, Nobuhiko Ohno3,4, Mio Inoue1, Mai Kagamiuchi1, Daisuke Kato1, Mami Matsumoto5,6, Yoshihiro Hasegawa1, Junichi Nabekura7,13, Anne Schaefer8,9, Andrew J Moorhouse10, Takeshi Yagi2, and Hiroaki Wake1,11,12,13,14*
(*Corresponding author)
1. Department of Anatomy and Molecular Cell Biology, Nagoya University Graduate School of Medicine, Nagoya, 466-8550, Japan.
2. KOKORO-Biology Group, Laboratories for Integrated Biology, Graduate School of Frontier Biosciences, Osaka University, Osaka, 565-0871, Japan.
3. Department of Anatomy, Division of Histology and Cell Biology, Jichi Medical University, Tochigi, 329-0498, Japan.
4. Division of Ultrastructural Research, National Institute for Physiological Sciences, Okazaki, 444-8585, Japan.
5. Section of Electron Microscopy, Supportive Center for Brain Research, National Institute for Physiological Sciences, Okazaki, 444-8585, Japan.
6. Department of Developmental and Regenerative Neurobiology, Institute of Brain Science, Nagoya City University Graduate School of Medical Sciences, Nagoya, 467-8601, Japan.
7. Division of Homeostatic Development, National Institute for Physiological Sciences, Okazaki, 444-8585, Japan.
8. Center for Glial Biology, Department of Neuroscience and Psychiatry, Friedman
Brain Institute, Icahn School of Medicine at Mount Sinai, New York, 10029-6574, US.
9. Max Planck Institute for Biology of Ageing, Cologne, 50931, Germany
10. School of Biomedical Sciences, The University of New South Wales, Sydney, 2052, Australia.
11. Division of Multicellular Circuit Dynamics, National Institute for Physiological Sciences, Okazaki, 444-8585, Japan.
12. Center for Optical Scattering Image Science, Kobe University, Kobe, 657-8501, Japan.
13. Department of Physiological Sciences, Graduate University for Advanced Studies, SOKENDAI, Hayama, 240-0193, Japan.
14. Core Research for Evolutional Science and Technology, Japan Science and Technology Agency, Saitama, 332-0012, Japan.
DOI:10.1016/j.celrep.2023.112383
5251