Key Points
○運動学習により
・Motor learning dynamically induces changes in lipid synthesis of the white matter beneath the motor cortex.
・Changes in lipid synthesis positively correlated with the intensity of neural activity associated with motor learning.
・Inhibition of galactosylceramide synthesis in oligodendrocytes impairs motor learning.
A research group led by Yuki Aoyama, PhD student, Daisuke Kato, Lecturer, and Hiroaki Wakei, Professor, at the Department of Anatomy and Molecular Cell Biology, Nagoya University Graduate School of Medicine, has focused on the myelin sheath, which is formed to insulate the axon, the output part of neurons in the brain. They found that changes in neural activity induced by motor learning result in alterations in the composition of lipids, a component of the myelin sheath, which are essential for motor learning, and the detailed mechanisms underlying these changes.
The brain consists mainly of grey matter, which is composed of neurons and their connecting synapses, and white matter, which is composed of axons (output fibers) and the myelin sheath surrounding them. Each of these acts like a computer and its cables, in which there are neurons that transmit information by electrical signals and glial cells that support their function. One type of glial cell, the oligodendrocyte, forms a myelin sheath around the axon that regulates conduction velocity. This myelin sheath is known to be a lipid-rich structure, which gives the white matter a white (lipid) color and allows it to act as an insulator. In recent years, plastic changes in the myelin sheath have been shown to alter the propagation of action potentials transmitted in the cable, which in turn contributes to the communication between neurons. In addition, studies using human head Magnetic Resonance Imaging (MRI) have reported that training, such as piano playing or juggling, causes structural changes in the white matter of relevant brain regions, suggesting that these changes occur in a neural activity-dependent manner induced by these trainings and that these changes are necessary for skill acquisition. Furthermore, head MRI studies in rodents have revealed that this plastic structural change in the white matter correlates with the expression of myelin-related proteins, thus focusing attention on the mechanisms responsible for the plastic structural change in the myelin sheath.
In this study, we focused on the lipid components of the myelin sheath and showed that these contribute to motor learning by revealing detailed changes in lipid composition during motor learning. It is hoped that this research will lead to the development of therapeutic strategies for Alzheimer's disease and multiple sclerosis, where the pathogenesis is known to progress in association with changes in the myelin sheath.
This research was conducted in collaboration with Professor Mitsutoshi Seto of Hamamatsu University School of Medicine, Assistant Professor Shiori Goh and Professor Kenji Kadomatsu of Institute for Glyco-core Research, Nagoya University, Professor Ikuko Yao of Kwansei Gakuin University and Dr Andrew J Moorhouse of the University of New South Wales.
This work was published online in GLIA on July 20, 2023. |
1. Research Background
The brain is made up of grey and white matter. This white matter is a cable of oligodendrocytes forming an insulating myelin sheath around axons, which plays an important role in facilitating information transmission between grey matte by increasing the propagation of action potentials and providing energy through the myelin sheath (Figure 1). Recent head MRI studies have also shown that the structure of the myelin sheath and its components, in particular the white matter, changes in response to the intensity of neural activity, and especially the increased expression of myelin-related proteins in specific brain regions is known to be significantly related to motor learning and skill acquisition. Therefore, most previous studies on neural activity and myelin sheaths have focused on learning-induced expression of myelin-related proteins, and little is known about learning-induced changes in lipid composition, a major component of the myelin sheath, and its associated contribution to neural activity.
In this study, we investigated whether changes in lipid composition, another possible aspect of plastic changes in the myelin sheath, are associated with motor learning. To this end, we combined a motor learning task in mice with two-photon Ca2+ imaging of neural activity in the primary motor cortex (M1) to quantify neural activity associated with learning. We then quantified the major lipid composition in the white matter beneath M1 by mass microscopy, which allows lipid composition to be examined on brain tissue sections while maintaining their spatial information. The results showed that sphingomyelin (SM) expression increased from early to middle stage of motor learning and galactosylceramide (GalCer) expression increased from middle to late stage of motor learning. Furthermore, the expression changes in these two lipid compositions were also higher in mice with greater learning performance and changes in learning-related neural activity. Finally, to test the causal relationship between motor learning and changes in the lipid composition of the myelin sheath, motor learning was performed while oligodendrocyte-specific inhibition of the expression of galactosyltransferase (CGT), an enzyme that synthesises GalCer. The results showed that motor learning was impaired, revealing that the lipid composition forming the myelin sheath changes dynamically in response to neural activity as part of the mechanism by which motor learning is improved.
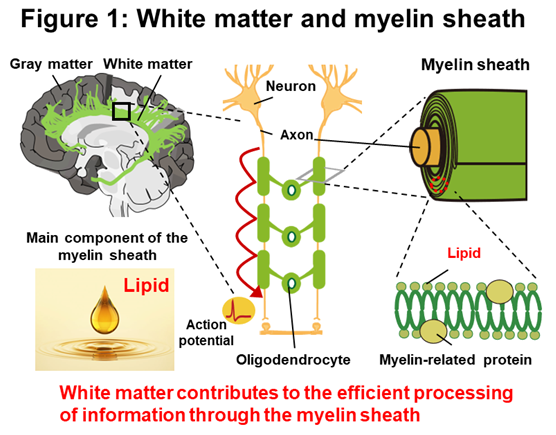
2. Research Results
The present study revealed the mechanisms of dynamic lipid synthesis in the myelin sheath during lever-pull motor learning and their contribution to improved lever-pull motor learning. Using approaches such as two-photon Ca2+ imaging, mass spectrometry microscopy, electron microscopy, electrophysiological, molecular biological and genetic methods (Figure. 2), the research group found that during the process of improved motor learning, (1) neural activity in M1 associated with successful lever pulling movements increases, (2) changes in the lipid composition of the white matter beneath M1 accompanying changes in neural activity promote the synthesis of SM and GalCer, and (3) inhibition of GalCer synthesis specifically by oligodendrocytes forming the myelin sheath impairs motor learning, indicating that GalCer synthesis is required for motor learning (Figure. 3).
(1) Changes in task performance and neural activity during the lever-pull motor learning
First, the research group used two-photon microscopy, which can observe the brains of live mice, to observe the neural activity of M1 mice over time during the lever-pull motor learning. The motor learning process was divided into three stages: early group (4 days of motor learning), middle group (7 days of motor learning) and late group (12 days of motor learning). By comparing the intensity and activity patterns of the same neurons, we quantified the learning efficiency and changes in neural activity in each group. The results showed that in M1 neurons, a number of neurons were observed to have activity associated with successful lever-pulling behavior (learning-related neural activity). In the early group, the mice with increased intensity of this learning-related neural activity performed more lever pulls. Furthermore, in the later group, mice with a greater number of neurons with increased intensity of learning-related neural activity (learning-related neurons) showed a higher success rate of lever pulls (higher learning efficiency). These results indicate that in the early stage of motor learning, M1 neural activity is induced for the lever-pull behavior, and in the later stage, this M1 neural activity changes to a lever-pull-specific neural activity pattern to increase learning efficiency.
(2) Changes in SM and GalCer expression in white matter beneath M1 correlate with neural activity during motor learning
Next, to investigate the myelin-specific changes in lipid composition that are altered during motor learning, we quantified the changes in lipid composition in the white matter beneath M1 using mass spectrometry microscopy. Brains were collected from the above three groups of mice in which motor learning and neural activity were individually measured, and the expression levels of SM and GalCer in the white matter beneath M1 on the left (motor learning side) and right (non-motor learning side) sides of the brain sections were measured and quantified using mass spectrometry microscopy. We found that SM expression was higher in the motor-learning side than in the non-motor-learning side in the early and middle groups, while GalCer expression was higher in the motor-learning side than in the non-motor-learning side in the middle and late groups. Furthermore, the results of LC-MS/MS and thin-layer chromatography on brain samples from the late group were also consistent with previous results.
In the early group, the expression levels of SM were found to correlate positively with the amount of increase in learning-related neural activity intensity during the learning process. Furthermore, in the late group, GalCer expression levels correlated positively with the proportion of neurons in which learning-related neural activity increased during the learning process. These results suggest that SM expression may contribute to increased neural activity associated with learning in the early stages of motor learning, while GalCer expression may regulate neural circuitry by promoting neural activity patterns that maintain success rates in the middle and late stages of motor learning.
(3) Inhibition of oligodendrocyte-specific GalCer synthesis reduces motor learning efficiency
Finally, we used RNA interference with adeno-associated virus to inhibit oligodendrocyte-specific CGT, as GalCer is synthesised from SM by the enzyme galactosyltransferase (CGT) in oligodendrocytes, and GalCer synthesis contributes to motor learning and synchronized neural activity. We found that oligodendrocyte-specific CGT inhibition reduced motor learning efficiency. In addition, focusing on the neural circuitry connecting the thalamus and M1, which is important for motor learning, we investigated the arrival time of action potentials transmitted through axons using electrophysiological techniques. The results showed that CGT inhibition significantly increased the variation in the arrival time of action potentials propagation in each axon. This suggests that oligodendrocyte-specific CGT inhibition may impair the timing of action potential propagation in individual axons connecting the thalamus and M1, thereby reducing motor learning efficiency, and thus GalCer synthesis in the myelin sheath is essential for motor learning.
3. Future Perspective
This study provides that GalCer synthesis helps stabilize neural activity associated with motor learning and that activity-dependent myelination mediated by changes in lipid composition is essential for the efficiency of motor learning. Recently, abnormalities in lipid metabolism in the myelin sheath have been associated with neurodegenerative diseases such as Alzheimer's disease. Furthermore, changes in lipid composition in the myelin sheath may contribute to the progression of ageing and neurodegenerative diseases, as imaired white matter function is often observed in the elderly and patients with neurodegenerative diseases. Therefore, the results of the present study suggest that regulating the synthesis of specific lipids in the myelin sheath may lead to new therapeutic strategies for diseases associated with impaired white matter function in the future.
4. Acknowledgements
This study was supported by the Grants in Aid for Transformative Research Areas (A) (20H05899 to H.W.); Fostering Joint International Research (B) (20KK0170 to H.W.); and AMED-CREST (JP23gm1410011 h0002 to H.W.).
5. Publication
Journal: GLIA
Title: Regulation of lipid synthesis in myelin modulates neural activity and is required for motor learning
Authors: Daisuke Kato1,2#*, Yuki Aoyama1#, Kazuki Nishida3, Yutaka Takahashi4, Takumi Sakamoto4, Ikuko Takeda1,2, Tsuyako Tatematsu1, Shiori Go5, Yutaro Saito1, Shiho Kunishima1, Jinlei Cheng1, Lingnan Hou1, Yoshihisha Tachibana3, Shouta Sugio1, Reon Kondo1, Fumihiro Eto4,6, Shumpei Sato4, Andrew J Moorhouse7, Ikuko Yao4,6, Kenji Kadomatsu5, Mitsutoshi Setou4 and Hiroaki Wake1,2,8,9,10*
# These two authors are designated as co-first authors.
* These two authors are designated as co-correspondence authors.
Affiliations:
1. Department of Anatomy and Molecular Cell Biology, Nagoya University Graduate School of Medicine, Nagoya, Japan.
2. Division of Multicellular Circuit Dynamics, National Institute for Physiological Sciences, National Institutes of Natural Sciences, Okazaki, Japan.
3. Division of System Neuroscience, Kobe University Graduate School of Medicine, Kobe, Japan.
4. Department of Cellular and Molecular Anatomy, Hamamatsu University School of Medicine, Hamamatsu, Japan.
5. Institute for Glyco-core Research, Nagoya University, Nagoya, Japan.
6. Department of Biomedical Chemistry, School of Science and Technology, Kwansei Gakuin University, Sanda, Japan.
7. School of Medical Sciences, UNSW Sydney, Sydney, NSW, Australia.
8. Center of Optical Scattering Image Science, Kobe University, Kobe, Japan.
9. Department of Physiological Sciences, Graduate University for Advanced Studies, SOKENDAI, Hayama, 240-0193, Japan.
10. Core Research for Evolutional Science and Technology, Japan Science and Technology Agency, Saitama, Japan.
DOI: 10.1002/glia.24441
5251